Specifically, sea ice motion data depicts sea ice dynamics, namely drift (transport) and deformation (gradients in sea ice motion; divergence/convergence capturing opening/closing associated with what are referred to as flaw leads and polynyas, in addition to rafting and ridging in the sea-ice cover). Forces that contribute to sea ice motion include winds on daily and weekly timescales, ocean currents on monthly and seasonal timescales and longer, the Coriolis force due to the Earth’s rotation, internal ice stress which measures ice strength determined by ice thickness (i.e. stronger/weaker ice is associated with thicker/thin ice), and sea surface tilt due to the undulating and dynamic ocean surface.
Ice motion is of high importance for activities on a short temporal horizon, including daily and weekly monitoring, forecasting and prediction. Specific applications include pollutant and contaminant transport, age of ice (tracking ice that survives the summer season), search and rescue, navigation (vessels can be trapped by moving ice floes) and planning for fishing and shipping routes and ice hazard detection in regions of construction (offshore structures can be damaged by the strength and momentum of moving ice) (Natural Resources Canada, 2021). Sea ice dynamics in the vicinity of coastal regions can contribute to sediment transport through advection following landfast ice break-up, and deformation where ice keels can redistribute shelf sediment (Barnhart et al., 2014). Recent development of SAR-derived sea ice drift on spatial scales of several kilometres enables landfast ice and stamukhi detection, relevant from the perspective of coastal erosion and permafrost degradation (Selyuzhenok and Demchev, 2021). For climate-related questions, realistic representation of sea ice dynamics from both an Eulerian and Lagrangian perspective is integral to reliable overall sea ice simulations. In particular, Lagrangian studies of sea ice drift (i.e. following ice floe trajectories) demonstrate a reduction in ice travel times with a transition from multi-year to perennial ice (Pfirman et al., 2004), with implications for freshwater transport and Arctic navigation (DeRepentigny et al., 2020). Eulerian studies of sea ice drift over several decades demonstrate acceleration in sea ice drift due to increased responsiveness of a weaker sea ice cover to surface winds, with implications for stratification and deep convection that would lead to a new CO2 sink (Hakkinen et al., 2008). More recently, age of ice studies using sea ice drift fields demonstrated a reduction of thick, multi-year ice north of Greenland in a region traditionally recognized as the last refuge for multi-year ice (Schweiger et al., 2021).
Historical Arctic sea ice motion observational data exist in the form of gridded (Eulerian; OSISAF, CERSAT, Polar Pathfinder) and Lagrangian (IABP) products. The following provides a brief overview of sea ice motion data of potential interest to data users in northern Canada, in addition to references comparing recent datasets and limitations. As for other sea ice variables, these datasets are featured due to their availability, decades-long duration, and local and global coverage; a subset is also used in deriving the global sea ice climate indicator of sea ice age.
name | source | data type | spatial domain | spatial resolution | temporal coverage | time step | data format | |
---|---|---|---|---|---|---|---|---|
IABP | University of Washington | Station data | irregular | Point data | 1979 to present | Variable ( sub-daily to daily) | ASCII; NetCDF | details |
Low Resolution OSISAFOSI-405 series | EUMETSAT | Satellite data | Global | 62.5 km x 62.5 km | 12/2009 – 07/2021 Summer data available following 2017 | Daily | NetCDF | details |
Medium-resolution OSISAFOSI-407 series | EUMETSAT | Satellite data | Global | 20 km x 20 km | 12/2009 – 07/2021 Summer data available following 2017 | 6h | NetCDF | details |
CERSAT | IFREMER | Satellite data | Global | 62.5 km x 62.5 km | 10/1999 – 05/2020 | Daily; Weekly; Monthly | NetCDF | details |
Polar Pathfinder 25 km daily EASE-grid sea ice motion vectors | NSIDC | Satellite data | Global | 25 km x 25 km | 25/10/1978 – 30/12/2020 | Daily; Weekly | PNG; NetCDF | details |
a) International Arctic Buoy Program (IABP) buoy data
Of interest at local spatial scales and high frequencies are sea ice beacon trajectories associated with the (Lagrangian) International Arctic Buoy Program (IABP) product. IABP data provides latitude and longitude coordinates recorded by buoys as direct measurement of ice floe movement and displacements (Figure 3.9). Sea ice motion vector components are computed by dividing beacon displacements in the zonal and meridional directions by elapsed time. IABP data are used both in an operational and research capacity. Operational applications include the use of beacon data in ice charts, to forecast weather and sea ice conditions (Rigor and Wallace, 2004), validate satellite forecasts, and incorporate in atmospheric reanalysis products to provide insight at local (several km) scales. Research applications include the use of beacon data to assess changes in sea ice drift and deformation in response to a changing climate over the past four decades, and to validate weather and climate models, as well as satellite-derived sea ice motion products, as is described in the next section.
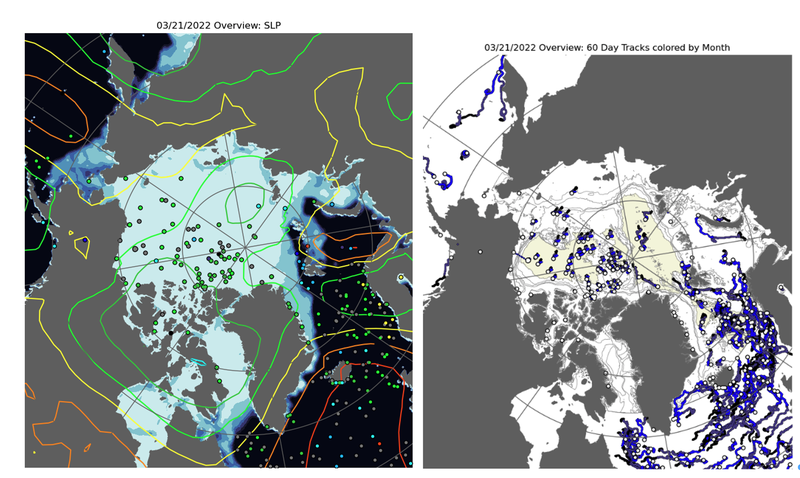
Figure 3.9. Example of (left) IABP sea ice beacon locations, NSIDC sea ice concentration, and NCEP SLP on March 21, 2022, and (right) 60 day tracks of sea ice buoy.
Considerations and Limitations
The IABP dataset is beneficial for assessments of local and regional sea ice drift and deformation for timescales ranging from several hours to months. By tracking ice floe movement, IABP data also captures small-scale features including loops, meanders, and eddies in response to atmospheric and oceanic forcing and ice strength at various locations and dates during the forty-year record. Furthermore, data is provided during summer months for the past four decades in contrast to satellite-derived ice motion data. However, spatial and temporal inhomogeneity in the beacon observations limit comparison of changes in ice dynamics for a given location over several decades, or regional differences in sea ice drift at a given instant or for a specific interval in time. Very few (if any) buoys reach waters outside of the Arctic Ocean (i.e. CAA, Hudson Bay, East Coast).
a) Satellite-Derived Sea Ice Motion
Gridded (Eulerian) sea ice motion products derived from satellite observations provide continuous spatial and temporal coverage of the Arctic over the past several decades. Sea ice motion is derived from a combination of passive microwave, scatterometer, radiometer, IABP buoy, and NCEP/NCAR wind data, using algorithms associated with the maximum cross-correlation technique that result in a daily merged product and a corresponding uncertainty.
Low-resolution OSI SAF Sea Ice Drift
The Ocean and Sea Ice Satellite Application Facility (OSISAF) EUMETSAT sea ice drift product is derived using a ‘continuous maximum cross correlation’ method applied to sub-images and designed to reduce tracking noise (Lavergene et al, 2010).. Uncertainties are also included for the x- and y- (2-day) displacements, and depict standard error. Yearly, hemispheric accuracy for the Arctic ranges from 2.5 km to 4.5 km for the single-sensor products, and approximately 2.6 km for the merged product. Only the multi-sensor product is appropriate for analysis during the summer months (May 1st to November 1st in the Northern Hemisphere), with reduced accuracy due to surface melting that hinders retrievals from instruments used to derive the ice-drift products. The x and y displacements, dx and dy (along the grid y-axis) are provided in units of km for the 2-day elapsed time intervals. The coarse resolution of this product (62.4 km), which captures regional scale sea ice motion, is not well resolved in small channels such as in the Canadian Arctic Archipelago.
NSIDC Polar Pathfinder 25 km sea ice drift, version 4
Trends and the global climate indicator of sea ice age (https://nsidc.org/data/NSIDC-0611/versions/4 ) can be respectively computed and derived from the decades-long Polar Pathfinder daily 25 km sea ice drift dataset, version 4, produced by the National Snow and Ice Data Centre (NSIDC). This product is derived from the merging of several datasets and sensors, including AVHRR, buoy, NCEP/NCAR, and passive microwave (SMMR, SSM/I, SSMIS, AMSR-E instruments) data using optimal interpolation and a cokriging estimation method. The final error variance in the merged sea ice motion product is based on a source- and distance-weighted average computed according to cross correlations between the individual sea ice motion estimate and buoy vector, and distance from the point being estimated (Tschudi et al., 2019). Differences between the interpolated and buoy components are on the order of 0.1 cm/s for the zonal component and 0.4 cm/s for the meridional component with root-mean-square errors of 3.36 cm/s and 3.4 cm/s respectively (Measuring sea ice motion, NSIDC, https://nsidc.org/sites/nsidc.org/files/technical-references/MeasureSeaIceMotion-0116-0748.pdf ).
Considerations and Limitations
Sumata et al. (2014) provide a comprehensive low-resolution product description and inter-product comparison for satellite-derived sea ice motion data. Here it is shown that larger error in satellite-derived sea ice drift is associated with higher drift speeds, and in regions of low sea ice concentrations and thickness. Inter-product comparisons further demonstrated maximum differences in ice-drift speeds on the order of 12% in Fram Strait, and in sea ice deformation on the order of 24% in winter and 37% in summer in the Amerasian Basin. It was also highlighted that all products, with suitable uncertainty estimates, were appropriate for sea ice dynamics (drift and deformation) model validation and data assimilation, with NSIDC and OSISAF showing lower uncertainties than CERSAT due to low ice drift speed bias in the latter. Cloud cover also hinders sea ice detection, resulting in missing data. Vector fields for all products are less accurate during summer than for fall, winter, or spring. The coarse resolution of this product for regional scale sea ice motion is not well resolved in small channels such as in the Canadian Arctic Archipelago.
As for atmospheric data products, discontinuities exist in sea ice drift data due to the merging of multiple data sources over a range of spatial and temporal scales. This is manifest in the NSIDC sea ice motion data set as circular, persistent features associated with the merging of buoy with satellite data.
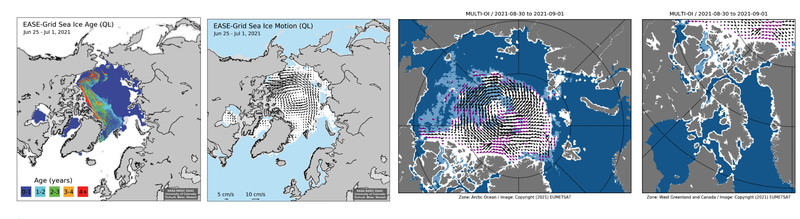
Figure 3.10 Examples of NSIDC (left) sea ice age derived from (middle) Polar Pathfinder 25 km sea ice motion from June 25 – July 1, 2021, and (right) OSI SAF sea ice drift for August 31, 2021 based on time elapsed from August 30 to September 1, 2021. [Source: https://daacdata.apps.nsidc.org/pub/DATASETS/nsidc0749_ql_iceage/,
https://daacdata.apps.nsidc.org/pub/DATASETS/nsidc0748_ql_icemotion/,
https://osisaf-hl.met.no/quicklooks-1prod?year=2021&month=09&day=01&prod=LR-Drift&time=Daily&area=NH]
References - Sea Ice Drift
Barnhart, K.R., I. Overeem, and R.S. Anderson, 2014: The Effect of Changing Sea Ice on the Physical Vulnerability of Arctic Coasts. The Cryosphere, 8(5), 1777-1799, doi:10.5194/tc-8-1777-2014.
DeRepentigny, P., A. Jahn, B. Tremblay, R. Newton, and S. Pfirman, 2020: Increased Transnational Sea Ice Transport Between Neighboring Arctic States in the 21st Century. Earth's Future, 8(3), doi.org/10.1029/2019EF001284.
Hakkinen, S., A. Proshutinsky, and I. Ashik, 2008: Sea Ice Drift in the Arctic Since the 1950s. Geophysical Research Letters, 35(19), L19704, doi:10.1029/2008gl034791.
Lavergne, T., S. Eastwood, Z. Teffah, H. Schyberg, and L.-A. Breivik, 2010: Sea Ice Motion from Low Resolution Satellite Sensors: An Alternative Method and Its Validation in the Arctic. Journal of Geophysical Research: Oceans, 115(C10), doi.org/10.1029/2009JC005958.
Lund, B., H.C. Graber, P.O.G. Persson, M. Smith, M. Doble, J. Thomson, and P. Wadhams, 2018: Arctic Sea Ice Drift Measured by Shipboard Marine Radar. Journal of Geophysical Research: Oceans, 123, 4298-4321, doi.org/10.1029/2018JC013769.
Natural Resources Canada, 2021: Ice motion, Retrieved from: https://www.nrcan.gc.ca/maps-tools-and-publications/satellite-imagery-and-air-photos/tutorial-fundamentals-remote-sensing/educational-resources-applications/sea-ice/ice-motion/9385.
Pfirman, S., W.F. Haxby, R. Colony, and I. Rigor, 2004: Variability in Arctic Sea Ice Drift. Geophysical Research Letters 31, doi:10.1029/2004GL020063.
Schweiger, A.J., M. Steele, J. Zhang, G.W.K. Moore, and K.L. Laidre, 2021: Accelerated Sea Ice Loss in the Wandel Sea Points to a Change in the Arctic’s Last Ice Area. Communications Earth & Environment, 2(1), doi:10.1038/s43247-021-00197-5.
Selyuzhenok, V., and D. Demchev, 2021: An Application of Sea Ice Tracking Algorithm for Fast Ice and Stamukhas Detection in the Arctic. Remote Sensing (Basel, Switzerland), 13(18), 3783, doi:10.3390/rs13183783.
Sumata, H., T. Lavergne, F. Girard-Ardhuin, N. Kimura, M.A. Tschudi, F. Kauker, M. Karcher, and R. Gerdes, 2014: An Intercomparison of Arctic Ice Drift Products to Deduce Uncertainty Estimates. Journal of Geophysical Research: Oceans, 119, 4887– 4921, doi:10.1002/2013JC009724.
Sumata, H., R. Kwok, R. Gerdes, F. Kauker, and M. Karcher, 2015: Uncertainty of Arctic Summer Ice Drift Assessed by High-Resolution SAR Data. Journal of Geophysical Research: Oceans, 120(8), 5285-5301, doi:10.1002/2015JC010810.
Sumata, H., R. Gerdes, F. Kauker, and M. Karcher, 2015: Empirical Error Functions for Monthly Mean Arctic Sea‐Ice Drift. Journal of Geophysical Research: Oceans, 120(11), 7450-7475, doi: 10.1002/2015JC011151.
Szanyi, S., J.V. Lukovich, D.G. Barber, and G. Haller, 2016: Persistent Artifacts in the NSIDC Ice Motion Data Set and their Implications for Analysis. Geophysical Research Letters, 43(20), 10,800-10,807, doi:10.1002/2016GL069799.
Tandon, N.F., P.J. Kushner, D. Docquier, J.J. Wettstein, and C. Li, 2018: Reassessing Sea Ice Drift and Its Relationship to Long‐Term Arctic Sea Ice Loss in Coupled Climate Models. Journal of Geophysical Research: Oceans, 123(6), 4338-4359, doi:10.1029/2017jc013697.
Thorndike A.S., 1986: Kinematics of Sea Ice. The Geophysics of Sea Ice, NATO ASI Series (Series B: Physics), Springer, Boston, MA, doi.org/10.1007/978-1-4899-5352-0_8.
Tschudi, M., W.N. Meier, J.S. Stewart, C. Fowler, and J. Maslanik, 2019: Polar Pathfinder Daily 25 km EASE-Grid Sea Ice Motion Vectors, Version 4. Boulder, Colorado USA, NASA National Snow and Ice Data Center Distributed Active Archive Center, doi.org/10.5067/INAWUWO7QH7B.